Perspectives for Design and Implementation
Downloads
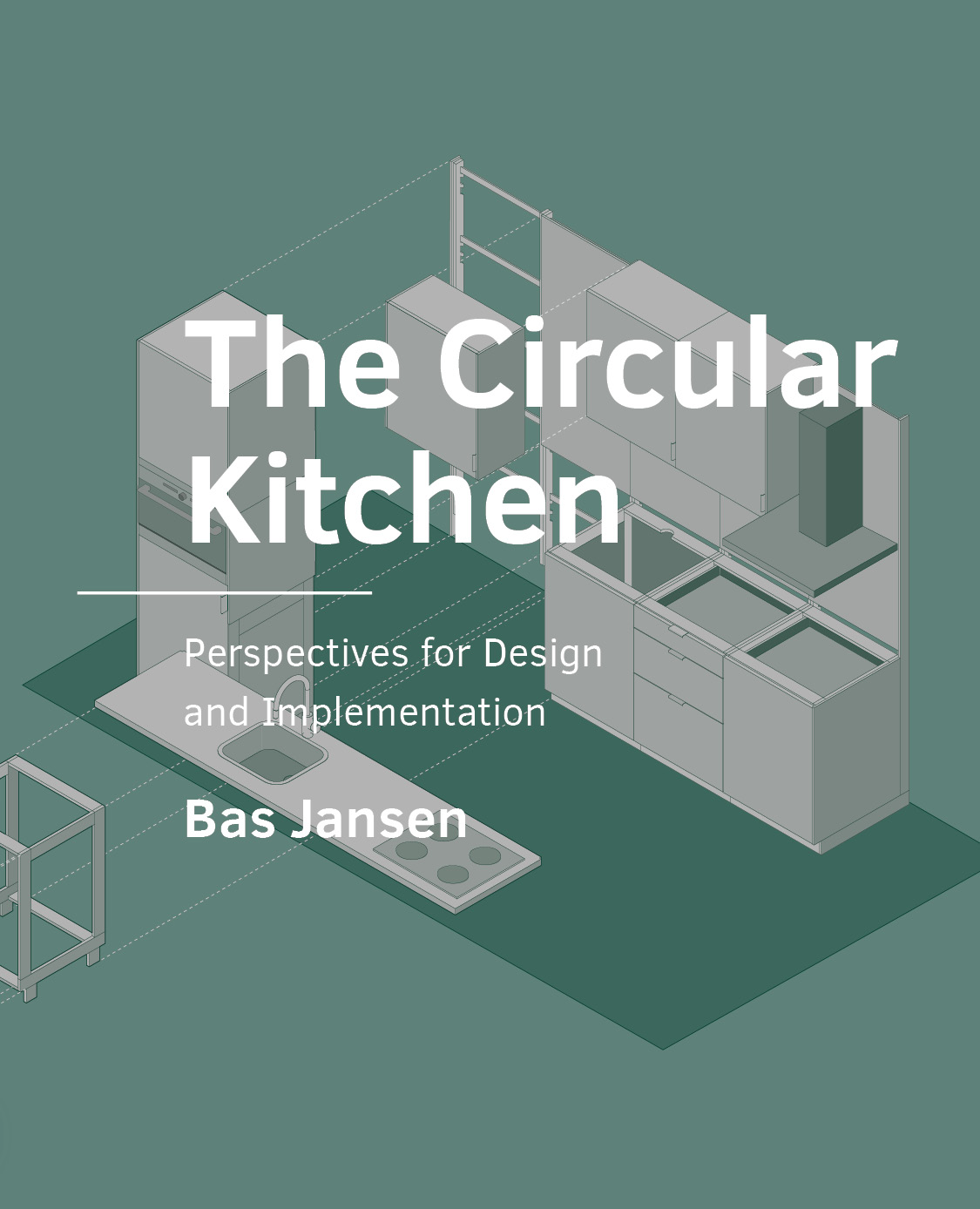
Published
Issue
Section
License
Copyright (c) 2025 Bas Jansen

This work is licensed under a Creative Commons Attribution 4.0 International License.
How to Cite
Abstract
Introduction
Recent reports emphasize the dire consequences of global warming exceeding 1.5°C, including ecosystem destruction and a fourfold increase in economic impacts by 2100. Despite housing being essential, the building sector’s substantial greenhouse gas emissions and material consumption necessitate an urgent transition to more sustainable approaches in the built environment to mitigate environmental and economic risks.
The Circular Economy (CE) presents a viable approach for achieving a sustainable built environment by minimizing resource use, environmental impacts, and waste. Geissdoerfer et al. (2017, p. 759) state that the CE is “a regenerative system in which resource input and waste, emission, and energy leakage are minimized by slowing, narrowing, and closing material and energy loops”. Slowing loops means to extend the lifespan of a building, a building component (such as a façade or roof), subcomponent (such as a window frame including glazing) or part (such as glazing); narrowing loops is to reduce resource use or enhance resource efficiency; closing loops is to recycle materials from end-of-life back to production (Bocken et al., 2016).
The Netherlands aims for a ‘fully circular’ economy by 2050, with a 50% reduction in primary resource use by 2030 (Ministerie van Infrastructuur en Milieu & Ministerie van Economische Zaken, 2016). Government-led initiatives and collaborations with industry stakeholders, such as the 2017 nationwide agreement to accelerate the transition to the circular economy (VNO-NCW et al., 2017) and subsequent transition agendas and roadmaps (Transitieteam Circulaire Bouweconomie, 2018, 2022), are driving the transition towards a circular built environment. This alignment between governmental policies and stakeholder interests creates an enabling environment for innovation and the advancement of circular practices.
Within that context, the housing sector could benefit the most from becoming more circular. Residential buildings, constituting over 87% of Dutch real estate (CBS, 2023a), face urgent renovation needs to reduce energy use, and an estimated one million homes need to be constructed to meet housing demand driving substantial embodied environmental impacts (Ministerie van Binnenlandse Zaken en Koninkrijksrelaties, 2022a, 2022b). Therefore, stricter regulations and circular economy principles are becoming central to new construction, renovation, and maintenance (Kamerbrief over Beleidsagenda Normeren En Stimuleren Circulair Bouwen, 2022). Housing associations manage a significant portion of Dutch homes, and are crucial in promoting circular practices (CBS, 2023b). Their substantial demand provides incentives for supply chain partners to develop circular alternatives, and their long investment perspective makes them well-suited for implementing CE principles.
Integrally developing circular building components
Authors such as Bocken et al. (2016), Mendoza et al. (2017), Pomponi and Moncaster (2017), Saidani et al. (Saidani et al., 2017), and van Stijn (2023) emphasize the importance of a comprehensive approach to circular design, urging consideration of the physical design, business model, and supply chain. Additionally, they argue that achieving circular design requires a systems approach, encompassing the entire design system from macro to micro levels.
However, achieving circularity at the city, or even building system level within a limited research timeframe is challenging. Hence, a building component approach is adopted. This approach, highlighted by researchers like van Stijn (2023) and Azcarate-Aguerre (2023), aims to narrow, slow, and close loops by substituting building components with circular alternatives during renovation, maintenance, or new construction. Specifically, this research focuses on developing a circular kitchen (the CIK) alongside other circular building components. While the environmental benefits of making a kitchen circular may be less significant than other components, the potential for widespread market adoption offers substantial overall environmental benefits due to kitchens’ low complexity, standardized production, and continuous demand for production.
Research on circular building components such as a circular kitchen involves exploring numerous design strategies, materials, and models for narrowing, slowing, and closing loops. Van Stijn & Gruis (2019) identified a vast array of design options for technical, industrial, and business models, resulting in millions of potential unique designs. However, not all designs may be environmentally or economically desirable or feasible. Despite the rise in research on circular economy (CE), its application in the built environment remained nascent, with a limited understanding of which designs offer the best environmental and economic performance and which designs are feasible in a real-world context.
Dissertation Goals
To address the gaps identified in the previous section, this research has a design goal and research goals. The development of the circular building component aimed to address the absence of such components in practice. The research goals were designed to support the development of the CIK while also generating valuable methods and knowledge.
The design goal is to develop a Circular Kitchen that is feasible in practice and performs better environmentally than non-circular kitchens. The focus is on implementing the CIK within Dutch Housing Associations (HAs) due to the favorable context for circular component development in the Netherlands, but the potential applicability and knowledge gained could apply to broader sectors.
Four research goals were identified to support the design goal while simultaneously developing methods applicable to other circular building components. First, this research aims to create circular kitchens that are environmentally superior to noncircular ones while remaining feasible. Economic viability is crucial for feasibility, thus an life cycle costing (LCC) method is developed. Research goal 1 (RG1) is therefore to develop an LCC method that determines the economic performance of circular building components. Second, the identification of the best-performing circular building component variants, encompassing both environmental and economic aspects, is essential for decision-making in the CIKs design. Research goal 2 (RG2) is, therefore, to identify which types of circular building component variants perform best environmentally and economically. Third, many authors have studied barriers and enablers for a circular built environment. However, to effectively address these barriers, decision-makers require a deeper understanding of their relative importance, their occurrence in real-world cases, and their impact on component feasibility. Such knowledge can inform better design, policy-making, and decisionmaking processes. Therefore, the third research goal (RG3) is to draw lessons from stakeholders’ choices in the CIK development that can aid the future development of feasible circular building components. Finally, the CIK is developed within a research context, which may lead to different design outcomes compared to kitchens developed outside of a research context. Analyzing other circular kitchens can yield insights into their feasibility and validate choices made during the development of the CIK, aligning with the fourth research goal (RG4), which is to identify which types of circular kitchens are feasible in practice, and examine their similarities and differences with each other and the CIK.
Approach and Methods
The research approach combines Research-through-Design (RtD) and Action Research (AR), involving cycles of designing and assessing circular kitchen designs. This Action Research-through-Design (ARtD) approach engages stakeholders in developing and testing the CIK, aiming to address real-world challenges while contributing to knowledge acquisition and implementation of the CE in the built environment.
The research commenced with a design process that raised numerous questions. Some of these questions found answers within existing literature, while others lacked relevant theories. Four research questions emerged from these inquiries, and these questions formed the basis of the research goals. Given the diversity of these research goals, the research methods employed varied per goal. Nevertheless, the overarching approach remained consistent.
A systematic design approach structured the development and testing of circular building components. It involved phases such as ‘proof-of-concept,’ ‘prototype,’ ‘demonstrator,’ and ‘market implementation,’ each encompassing analysis, synthesis, simulation (or test), and evaluation activities. Although deviations from the planned process necessitated multiple iterations and adjustments, this approach enhanced understanding and facilitated progress.
Results
Results research goal 1
In this study, three existing LCC approaches were identified: Conventional LCC (C-LCC), Environmental LCC (E-LCC), and Societal LCC (S-LCC). While C-LCC typically has a single stakeholder perspective and may overlook end-of-life scenarios, E-LCC broadens the perspective to include multiple stakeholders, and S-LCC considers both direct and indirect costs to society. However, existing methods do not fully incorporate the complex, multiple use cycles inherent in circular products and components.
To support the development of circular products, existing LCC techniques were adapted to (1) consider products as composite entities with varying use cycles, (2) include post-use processes, (3) offer practical information to stakeholders, and align functional units and system boundaries with life cycle analysis (LCA). The developed CE-LCC method (see Figure Sum.1) was applied to compare CIK variants, with the most adaptable variant showing the most favorable long-term LCC outcome. This model aids decision-makers in assessing the economic viability of circular products and thus supports the transition towards sustainability in the building industry.
Results research goal 2
This study aimed to assess the circular performance of building components, comparing biological (BIO) and technical (TECH) circular pathways in terms of environmental and economic performance. Circular economy life cycle assessment (CE-LCA)(expressed in shadow costs), material flow analysis (MFA), and CE-LCC (expressed in total costs (TC)) were used to compare CIK and circular façade design variants. Rankings were provided based on the outcomes for business-as-usual (BAU), BIO, TECH, and hybrid (HYBRID) design variants (see Table Sum.1). Results revealed that BIO solutions performed best in terms of shadow costs but ranked lower in MFA and TC, while some TECH solutions showed the opposite trend. HYBRID variants demonstrated potential for improved performance by combining BIO and TECH materials.
Importantly, BAU components consistently ranked lower than circular variants, suggesting the potential for enhancing environmental and economic performance through circular pathways. The study emphasized the need to apply materials and circular design principles effectively, aiming to mitigate environmental impacts, extend lifespan, and introduce multiple future cycles for components, parts, and materials. This reinforces the importance of transitioning to circular building components for a more sustainable built environment.
Results research goal 3
This study focused on identifying choices that stakeholders made toward a feasible circular design and the impact of those choices, aiming to support designers, policymakers, and decision-makers in other circular design processes. A longitudinal case study of a circular building component, the CIK, was conducted. The researchers actively co-created the CIK’s design, which is shown in Figure Sum.2, its supply chain model, and its business model throughout five phases, documenting all decisions made by stakeholders. Five lessons were derived by analyzing these stakeholders’ decisions and reflecting on the development process.
Lesson one emphasized the importance of prioritizing feasible circular design options over more ideal circular options, as the immediate implementation of circular solutions is more beneficial to a more sustainable built environment than postponing the implementation to create a ‘more circular’ design. The second lesson underscored the significance of component aesthetics for broad acceptance among clients and end-users, highlighting the need for satisfying various preferences. The third lesson stressed the substantial impact of decisions made at the detail scale on a component’s feasibility and circularity, recommending simultaneous design at different scales. The fourth lesson emphasized the importance of participation of stakeholders that are representative of the whole supply chain in aligning the value proposition and ensuring effective project focus. It suggested involving individuals with optimal influence, technical knowledge, and project dedication. The fifth lesson revealed the need for sufficient time and resources when considering integral redevelopment of the physical design, supply chain model, and business model.
While these lessons may not cover all contexts comprehensively, they offer insights into decision-making during circular component development, potentially aiding future component development.
Results research goal 4
In this study, seven circular kitchens, including the CIK, were identified and compared to identify which types of circular kitchens are feasible in practice. These kitchens can be seen in Figure Sum.3a-g. These kitchens were manufactured by both established companies and start-ups, revealing differences in the degree of innovation applied. The established manufacturers tended to align more closely with non-circular kitchen models, while start-ups implemented more radical innovations. Detailed information was primarily available concerning the technical model, while insights into industrial and business models were relatively scarce.
Notably, the kitchen designs displayed a bifurcation based on material choices for their structure, specifically between frame structures using technical materials (in this case steel) and panel-based structures using biological materials (different types ranging from plywood to cellulose panels). The CIK stood out with its biobased frame structure, which was later adapted by the manufacturer, indicating its infeasibility. A distinction was also noted regarding the use of retaining walls, a feature present in both frame and panel-based kitchens.
All of the examined circular kitchens prioritized circular design options to facilitate closing future loops, thereby enhancing their long-term environmental performance. However, it is crucial to acknowledge that the feasibility of circular kitchen types may change over time, and the absence of certain types in current practice does not necessarily signify their infeasibility. Additionally, since these kitchens were recently developed and none have reached their end-of-life stage, the extent to future resource loops will be closed and the actual benefits they will yield remains uncertain.
This study, while specific to the Dutch housing sector, provides valuable insights into feasible circular kitchen technical models. Such knowledge can ease the implementation of future circular kitchens, potentially streamlining industry-wide standardization and enhancing the circular transition. The study also highlights the disparity between ideal and feasible circular designs in a research context, and circular kitchens in practice, emphasizing the importance of considering market implementation in such projects.
Conclusions
The building industry plays a critical role in advancing sustainability, with a shift to a Circular Economy (CE) offering the potential for reducing resource consumption and waste. Circular kitchens stand out as promising options due to their simplicity in manufacturing, existing standardization, streamlined supply chains, and controlled indoor usage, minimizing implementation risks. Various strategies can be employed to develop circular building components like kitchens, aiming to narrow, slow, or close resource loops.
This research aimed to a feasible Circular Kitchen (CIK) that reduces environmental impacts compared to current, non-circular kitchens, targeting housing associations as primary clients due to their significant market presence and long-term investment strategies. To achieve this, four research goals (RGs) were defined: developing an LCC method for economic evaluation of circular components (RG1), assessing the environmental and economic performance of circular building components (RG2), deriving lessons from stakeholders’ choices in CIK development (RG3), and investigating the feasibility of circular kitchens beyond the CIK project (RG4).
So, was the goal of developing such a circular kitchen reached? Was the CIK feasible in practice, and did it perform better environmentally than current, non-circular kitchens? And if not, which strategies would be advisable to develop a better circular kitchen?
Conclusions on the design goal
All evaluated CIK designs outperformed standard non-circular kitchens economically and environmentally. The last design variant of the CIK, the TECH kitchen in Study 2, achieved the highest MFA performance and second-highest in CE-LCA. Conversely, the BIO kitchen excelled in CE-LCA and ranked second in MFA. While BIO designs share similarities with non-circular kitchens, implementing TECH designs requires substantial supply chain and business model changes. Insecurity regarding these changes potentially impacts environmental outcomes negatively. Biological solutions offer greater assurance regarding their environmental performance due to their impact reduction taking place early in the lifecycle, independent of future adoption of value retention processes (VRPs), such as reuse, remanufacturing, and recycling. Economic feasibility is paramount, with stakeholders emphasizing cost minimization and price parity with non-circular kitchens. Despite higher initial costs, the most adaptable CIK variant proved cost-effective over extended periods, confirmed by the second study comparing TECH and BIO kitchens. However, real-world feasibility was limited, as observed in studies three and four. Housing associations still prioritize total costs sparingly, and mass-producing CIKs necessitates significant investments in new production facilities. Additionally, the decision to make these investments coincided with unforeseen negative feedback received after placing demonstrator kitchens in several homes. Consequently, the CIK failed to transition beyond the research phase. In conclusion, the design goal was not reached, and the CIK did not reach application in the real world. Nevertheless, knowledge gained from the complete CIK research project were utilized to further develop a different, less ambitious circular kitchen design that is aimed to be implemented soon.
Reflections
This research comprehensively investigated circular kitchens across four studies, offering insights that extend beyond individual findings. This concluding section, therefore, presents three insights: improving the feasibility of circular kitchens, designing for a circular built environment, and improving the CE’s role in achieving sustainability. These reflections offer implications for practice, as well as directions for further research.
Reflections on better, more feasible circular kitchens
As can be concluded from the Section "Conclusions on the design goal", the CIK design was not feasible in the context that it was envisioned in. But how could a circular kitchen be feasible in this context? And how could that feasible circular kitchen still yield better environmental performance than the current standard kitchen? xxxxxx Study four shows the diverse approaches taken by start-ups and established manufacturers in developing circular kitchens. While start-ups favor radical innovation, established manufacturers, like the CIK’s producer, opt for incremental improvements (outside of a research context). This suggests that when aiming for large-scale implementation that can only be reached with large-scale production, the feasibility of the design should be prioritized, while still improving environmental performance compared to current, non-circular kitchens. Feasibility is achieved by reducing initial costs and aligning production methods and supply chains with current industry standards. Enhancing environmental performance involves applying low-impact or longer-lifespan materials, slowing potential loops through standardization, modular design, and demountable joints, and facilitating repairs and replacements where possible for minimal additional costs.
For manufacturers focused on gradual growth rather than immediate large-scale market adoption, radical innovation towards optimal environmental performance becomes viable. This entails narrowing material loops upfront, slowing and closing future loops through various design strategies. Design decisions can be based on the feasibility of total costs, and designs can apply production methods, business models, and supply chains that differ from the current industry standard. However, sustaining production, sales, reuse, and recycling is crucial, with ongoing efforts directed at improving financial viability to expand market share, and with it the environmental benefits.
Reflections on designing for a circular built environment
The production of standardized modular building components as products necessitates significant reconsideration of design methodologies from both product and building design perspectives. Although scholars of design theory acknowledge that many design phases tend to overlap or occur concurrently in practice (Milton & Rodgers, 2011), product and architectural design theories typically propose a design process with multiple subsequent phases (Ginting, 2020; Roozenburg & Eekels, 1995; Ulrich & Eppinger, 2008). In the first phases, the design is abstract (or global) and it progressively acquires more detailed specifications in subsequent phases – converging from global to detailed.
However, the third study of this dissertation showed that the successful design of modular building components relies heavily on decisions regarding connections, production methods, and materials that are conventionally made in the later phases of the design process. Therefore, the conventional design methods might not suffice, and an alternative approach is needed. This approach applies to both designing at the building and the component level.
Designers should determine the desired level of modularity early on and adapt the focus within the design process accordingly. A fully standardized modular building or component requires a detailed design approach from the start, diverging toward a global scale. Some examples of this approach can already be seen in the Dutch social housing sector, for instance in the newly built modular homes of the Bouwstroom (Aedes & VTW, 2022). Conversely, a fully custom building can follow the traditional converging design approach. Designs that neither follow a standardized modular approach, nor a completely custom approach fully, might require both the global and the detailed level as a starting point of the design process. Figure Sum.5 shows this proposal for such selection of design method based on the degree of standardized modularity. As is the case for conventional design phases, design iterations can go back and forth between phases – a design process is rarely a linear one – and this figure merely indicates one or multiple starting points for the design process, and a priority and general direction.
Reflections on slowing and closing loops
The CE offers potential solutions to reducing resource use, environmental impacts, and waste in the built environment, but blindly pursuing circularity may exacerbate environmental challenges. This research highlights the need to differentiate between circular strategies in fostering sustainability within the built environment. Study 2 reveals that not all circular design options improve environmental performance, sometimes even increasing resource use and waste generation. Additionally, circular components often require higher upfront investments, while only offering potential long-term benefits. Study 3 identifies challenges in developing components for future VRPs, requiring changes in business models, supply chains, and design methodologies. While feasible changes exist, they are mainly observed in small-scale production. Designing for future VRPs introduces uncertainty due to the long timespan in which they are beneficial environmentally, and their reliance on long-term stakeholder collaboration. Components relying on future VRPs may require multiple use cycles to achieve superior environmental performance, adding to the uncertainty of their benefits.
This research emphasizes the importance of prioritizing strategies with immediate environmental benefits due to the risks and challenges associated with achieving long-term benefits through building components designed for future Value Retention Processes (VRPs). Strategies such as Refuse, Rethink, and Reduce, along with substituting high-impact materials with low-impact, renewable ones and slowing and closing existing material loops, are crucial for consistently achieving a more sustainable built environment. In conclusion, not all circular approaches are beneficial to environmental goals in the short or long term, either due to their lack of environmental performance improvement or feasibility.
References
Aedes, & VTW. (2022). handreiking Conceptueel bouwen en bouwstromen.
Aguerre Azcarate, J. (2023). Facades-as-a-Service A cross-disciplinary model for the (re)development of circular building envelopes. https://doi.org/10.7480/abe.2023.11
Bocken, N. M. P., de Pauw, I., Bakker, C., & van der Grinten, B. (2016). Product design and business model strategies for a circular economy. Journal of Industrial and Production Engineering, 33(5), 308–320. https://doi.org/10.1080/21681015.2016.1172124
CBS. (2023a). StatLine - Voorraad woningen en niet-woningen; mutaties, gebruiksfunctie, regio. https://opendata.cbs.nl/statline/#/CBS/nl/dataset/81955NED/table?fromstatweb
CBS. (2023b). Voorraad woningen; eigendom, type verhuurder, bewoning, regio. https://www.cbs.nl/nl-nl/cijfers/detail/82900NED
Ginting, R. (2020). Integrated Model of Product Design Methods. In C. Alexandru, C. Jaliu, & M. Comşit (Eds.), Product Design (pp. 23–40). IntechOpen. https://mts.intechopen.com/storage/books/9174/authors_book/authors_book.pdf
Hensen, J., Loonen, R., Archontiki, M., & Kanelis, M. (2015). Using building simulation for moving innovations
across the “Valley of Death.” REHVA Journal, 58–62. www.peerplus.nl
Kamerbrief over Beleidsagenda Normeren En Stimuleren Circulair Bouwen (2022). https://www.cirkelstad.nl/wp3/wp-content/uploads/2022/06/Koplopers-in-Woningbouw-
Mendoza, J. M. F., Sharmina, M., Gallego-Schmid, A., Heyes, G., & Azapagic, A. (2017). Integrating Backcasting and Eco-Design for the Circular Economy: The BECE Framework. Journal of Industrial Ecology, 21(3), 526–544. https://doi.org/10.1111/jiec.12590
Milton, A., & Rodgers, P. (2011). Product design. In Z. Antoniou (Ed.), Product Design. Laurence King Publishing Ltd. https://learning-oreilly-com.tudelft.idm.oclc.org/library/view/productdesign/9781856697514/09_chapter04.html#c04-h1-001
Ministerie van Binnenlandse Zaken en Koninkrijksrelaties. (2022). Nationale Woon- en Bouwagenda. https://www.rijksoverheid.nl/documenten/rapporten/2022/03/11/nationale-woon-en-bouwagenda
Ministerie van Infrastructuur en Milieu, & Ministerie van Economische Zaken. (2016). Nederland circulair in 2050. https://open.overheid.nl/documenten/ronl-a6ce8220-07e8-4b64-9f3d-e69bb4ed2f9c/pdf
Pomponi, F., & Moncaster, A. (2017). Circular economy for the built environment: A research framework. Journal of Cleaner Production, 143, 710–718. https://doi.org/10.1016/j.jclepro.2016.12.055
Roozenburg, N. F. M., & Eekels, J. (1995). Product design : fundamentals and methods. In Product design: fundamentals and methods. Wiley.
Saidani, M., Yannou, B., Leroy, Y., & Cluzel, F. (2017). How to assess product performance in the circular economy? Proposed requirements for the design of a circularity measurement framework. Recycling, 2(1). https://doi.org/10.3390/recycling2010006
Transitieteam Circulaire Bouweconomie. (2018). Transitie-Agenda Circulaire Economie: Circulaire Bouweconomie.
Transitieteam Circulaire Bouweconomie. (2022). Adviesroute naar een circulaire economie voor de bouw.
Ulrich, K. T., & Eppinger, S. D. (2008). Product Design and Development (4th ed.). McGraw Hill. https://www.academia.edu/29638204/Product_Design_And_Development_4th_Edition_Ulrich
van Stijn, A. (2023). Developing circular building components. Between ideal and feasible. Delft University of Technology.
van Stijn, A., & Gruis, V. (2019). Towards a circular built environment: An integral design tool for circular building components. Smart and Sustainable Built Environment, 9(4), 635–653. https://doi.org/10.1108/SASBE-05-2019-0063
VNO-NCW, MKB-Nederland, FNV, VCP, Stichting Natuur & Milieu, VNG, IPO, Unie van Waterschappen, & Staatssecretaris van Infrastructuur & Milieu en de Minister van Economische Zaken. (2017). Grondstoffenakkoord.